Material Surface Treatment Process in the Automotive Industry
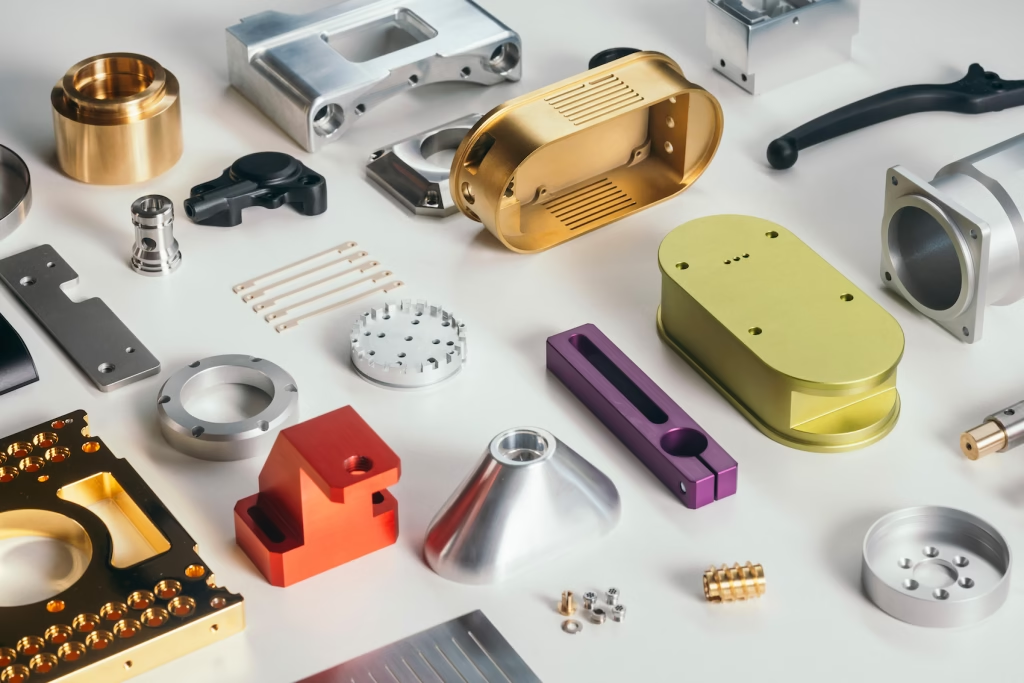
Material Surface Treatment Process in the Automotive Industry In the highly competitive automotive industry, the durability, performance, and aesthetic appeal of metal components are critical factors that influence product success. One essential step in ensuring these qualities is the material surface treatment process. Among the most widely used surface treatments in automotive manufacturing are nickel plating, zinc plating, black oxide, electropolishing, blasting, polishing, and anodizing. This article examines the primary surface treatment methods, their applications, and their significance in the automotive industry. What is the Material Surface Treatment Process? Material surface treatment refers to a series of techniques applied to the exterior layer of metals or alloys to alter their surface properties. The goal is to improve resistance to corrosion, wear, and fatigue, enhance appearance, or add special functionalities such as electrical conductivity or insulation. In automotive manufacturing, surface treatments ensure that parts withstand harsh environmental conditions, mechanical stress, and maintain aesthetic standards. Key Surface Treatment Processes in the Automotive Industry 1. Nickel Plating Nickel plating is an electrochemical process where a thin layer of nickel is deposited on a metal surface. This coating offers excellent corrosion resistance, hardness, and wear resistance, making it ideal for automotive components such as engine parts, fuel systems, and decorative trims. Process: A positively charged electrical current passes through a nickel salt solution, causing nickel ions to deposit on the negatively charged metal substrate. Benefits: Enhances durability, corrosion resistance, and provides an attractive, shiny finish. Automotive Use: Used in exhaust systems, fuel injectors, and various engine components. 2. Zinc Plating Zinc plating involves coating metal parts with a thin layer of zinc to prevent rust and corrosion, particularly for steel components. Process: Can be performed via electroplating or hot-dip galvanizing, where parts are dipped into molten zinc. Benefits: Acts as a sacrificial layer, protecting steel from oxidation. Automotive Use: Commonly applied to fasteners, body panels, and chassis parts to extend service life. 3. Black Oxide Black oxide is a chemical conversion coating that produces a thin, black protective film on steel surfaces. Process: Metal parts are immersed in an alkaline oxidizing solution, forming a magnetite (Fe3O4) layer. Benefits: Provides mild corrosion resistance, reduces light reflection, and improves lubricity. Automotive Use: Used on fasteners, brake components, and tools. 4. Electropolishing Electropolishing is an electrochemical process that smooths and brightens metal surfaces by removing microscopic peaks and burrs. Process: The metal part acts as an anode in an electrolytic bath, where surface atoms dissolve, resulting in a smoother finish. Benefits: Enhances corrosion resistance, reduces surface roughness, and improves cleanliness. Automotive Use: Applied to stainless steel exhaust parts, fuel systems, and precision components requiring a high surface finish. 5. Blasting Blasting refers to the mechanical cleaning or texturing of metal surfaces using abrasive materials. Types: Sandblasting, bead blasting, or shot blasting. Benefits: Removes rust, scale, and old coatings; prepares surfaces for further treatment. Automotive Use: Used for surface preparation before painting or coating on body panels and engine parts. 6. Polishing Polishing is a mechanical process that produces a smooth and shiny surface finish. Process: Uses abrasive compounds and polishing wheels to buff the surface. Benefits: Improves aesthetic appeal and reduces friction. Automotive Use: Applied to chrome trims, wheels, and interior metal parts. 7. Anodizing Anodizing is an electrochemical process that thickens the natural oxide layer on metals like aluminum. Process: Aluminum parts are submerged in an acid electrolyte bath while an electric current passes through, forming a durable oxide layer. Benefits: Enhances corrosion resistance, wear resistance, and allows for coloring. Automotive Use: Widely used for aluminum wheels, engine components, and decorative trims. Importance of Surface Treatment in the Automotive Industry The automotive industry demands components that can withstand extreme conditions such as temperature fluctuations, moisture, salt exposure, and mechanical wear. Surface treatments play a pivotal role in: Corrosion Resistance: Protecting metal parts from rust and degradation extends vehicle lifespan. Wear Resistance: Enhancing surface hardness reduces friction and wear in moving parts. Aesthetic Enhancement: High-quality finishes improve vehicle appearance and market appeal. Functional Improvements: Treatments like electropolishing improve cleanliness and reduce friction, critical for fuel efficiency and emissions. Environmental Compliance: Many surface treatments help meet environmental regulations by extending part life and reducing waste. Best Practices for Selecting Surface Treatments When choosing a surface treatment process for automotive components, consider: Material Compatibility: Ensure the treatment suits the base metal (steel, aluminum, stainless steel). Environmental Conditions: Select treatments that withstand specific exposures, like salt spray or heat. Functional Requirements: Prioritize treatments that enhance wear resistance, electrical conductivity, or aesthetics based on part function. Cost and Volume: Balance process costs with production scale and part complexity. Environmental Impact: Opt for eco-friendly processes where possible, such as electropolishing or powder coating. Conclusion The material surface treatment process is indispensable in the automotive industry, ensuring parts meet stringent performance, durability, and aesthetic standards. Techniques like nickel plating, zinc plating, black oxide, electropolishing, blasting, polishing, and anodizing each offer unique benefits tailored to specific applications. By integrating these surface treatments, automotive manufacturers can significantly enhance product quality, extend component life, and satisfy customer expectations. If you have any needs, contact us via email at Lynnyao@prototekparts.com or phone: +86-0792-86372550 Tags: Social Network: Recently Posts Online Help! +(86) 0791-86372550 WANT TO DISCUSS IN DETAIL Contact Our Consultant Now CONTACT NOW
Knowing the Key Valve Components
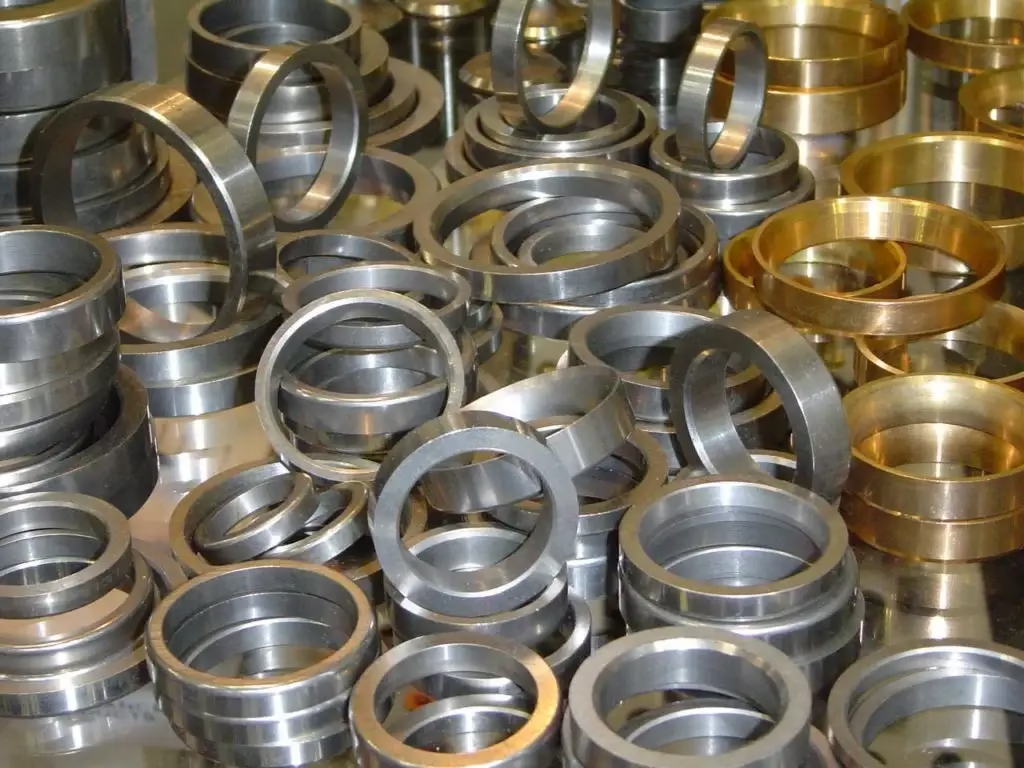
Knowing the Key Valve Components To maximize performance and guarantee dependability in the complex world of industrial valves, it is essential to comprehend the parts that comprise these essential devices. The valve seat, valve stem, and valve bonnet are some of the crucial parts. This article explores the roles and responsibilities of these parts, offering insights into their importance in the overall functioning of valves. Introduction In many industrial applications, valves are essential for regulating fluid flow and pressure. They are made up of a number of essential parts, each of which contributes in a different way to the safe and effective operation of the valve. The valve seat, valve stem, and valve bonnet are essential parts of this system. The Valve Bonnet: Protecting and Covering Its Features Functions of the Valve Bonnet A vital component of the valve’s construction and operation, the valve bonnet acts as the cover for the valve body. Its primary functions include: Pressure Containment: The bonnet acts as a seal, ensuring that the process fluid does not escape from the valve body. It is designed to withstand the maximum pressure of the valve’s intended use. Component Protection: By enclosing the internal parts of the valve, the bonnet protects them from external contaminants and damage, contributing to the valve’s durability and reliability. Access Point: The bonnet can serve as an access point for the maintenance, repair, or replacement of the valve’s internal components. Depending on the valve design, removing the bonnet may provide direct access to the internals. Types of Valve Bonnets Valve bonnets come in various designs, each suited to different applications and pressure conditions. Common types include: Bolted Bonnet: A widely used design where the bonnet is attached to the valve body with bolts or studs and nuts. It provides a sturdy seal and is suitable for a broad range of pressures and temperatures. Screwed Bonnet: In this design, the bonnet is screwed directly into the valve body. Screwed bonnets are commonly used in smaller valves and lower-pressure applications. Welded Bonnet: For valves used in high-pressure and high-temperature applications, a welded bonnet provides a leak-proof seal by permanently welding the bonnet to the valve body. Pressure Seal Bonnet: Used in high-pressure applications, this design employs a gasket that is pressure-energized to enhance the seal between the bonnet and the valve body as the internal pressure increases. Significance of the Valve Bonnet The valve bonnet plays a vital role in ensuring the safe and efficient operation of the valve. Its design and construction are critical for maintaining the integrity of the valve under operating conditions. Choosing the appropriate bonnet type based on the specific requirements of the application, such as pressure, temperature, and the need for maintenance access, is essential for valve performance and longevity. The Valve Stem: Connecting and Controlling Role of the Valve Stem The valve stem is a crucial component that connects the actuator or manual control mechanism to the valve disc or plug. It transmits the motion required to open or close the valve, playing a vital role in the valve’s operation. The stem’s movement can be linear, as in gate or globe valves, or rotational, as in ball, butterfly, or plug valves. Types of Valve Stems There are several types of valve stems, each designed for specific valve operations: Rising Stem with Outside Screw and Yoke (OS&Y): The external side of the stem is threaded while the part inside the valve is plain. The threads are isolated from the medium by the packing. Rising Stem with Inside Screw (IS&Y): The threaded part of the stem is positioned inside the valve body, whereas the stem packing lays outside. Non-Rising Stem with Inside Screw: The threaded part of the stem is inside the valve and does not rise. The valve disc floats on the stem, like a nut, if the stem is rotated. Importance of the Valve Stem The valve stem’s design and material are crucial for preventing leakages and ensuring smooth operation. A proper finish of the stem surface is necessary to prevent leakages, and the choice of material must withstand the operational conditions, such as pressure and corrosiveness of the medium. The Valve Seat: Sealing and Regulating Function and Importance of Valve Seats Valve seats are integral components of a valve’s internal mechanism, designed to interface with the valve disc to form a tight seal that controls or stops fluid flow through the valve. The primary functions of a valve seat include: Sealing Surface: Provides a smooth, precise surface for the valve disc to rest against, creating a seal that prevents fluid from leaking past the closed valve. Flow Regulation: In valves designed for throttling, the seat works in conjunction with the disc to modulate the flow of fluid. Durability and Wear Resistance: Valve seats must withstand continuous contact with the valve disc and resist wear and deformation from the process fluid, pressure fluctuations, and mechanical operations. Materials for Valve Seats Valve seats are made from a variety of materials, chosen based on the application’s specific requirements, including the type of fluid, operating pressure, temperature, and corrosive potential. Common materials include: Metals: Stainless steel, bronze, and brass are commonly used for their durability and resistance to high temperatures and pressures. Soft Seats: Materials such as PTFE (Teflon), nylon, and other elastomers offer excellent sealing capabilities and resistance to chemical corrosion. Ceramics: For high-wear or corrosive applications, ceramic seats offer superior hardness, wear resistance, and stability under extreme temperatures. Design Considerations The design of the valve seat is crucial for its function and longevity. Factors to consider include: Seat Shape and Angle: The contour and angle of the seat affect the sealing efficiency and flow characteristics. Interchangeability: Some valve designs feature replaceable seats, allowing for easy maintenance and extended valve life. Sealing Method: The seat-to-disc contact area and method of sealing (e.g., metal-to-metal, soft seal) determine the tightness of the seal and the valve’s suitability for specific applications. Conclusion The valve bonnet, valve stem, and valve seat are integral components
The Role of 5-Axis Machining in the Automotive Industry
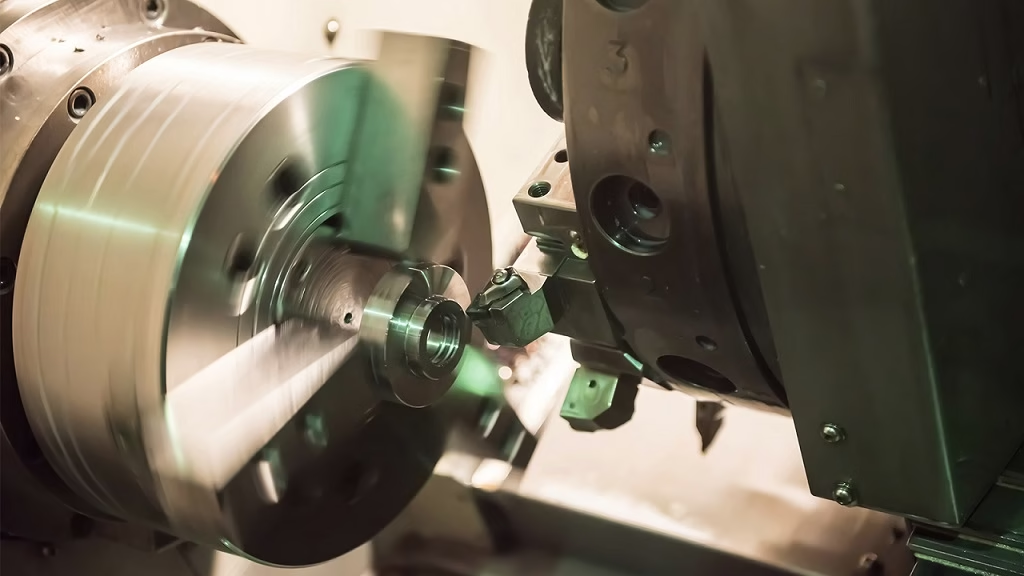
The Role of 5-Axis Machining in the Automotive Industry In the fast-paced world of manufacturing, precision and efficiency are paramount. One technology that has revolutionized the automotive industry is 5-axis machining. This advanced manufacturing technique allows for the creation of complex parts with unparalleled accuracy. In this article, we’ll delve into the intricacies of 5-axis machining, explore its application in the automotive industry, and highlight why manufacturers are increasingly adopting this technology. Understanding 5-Axis Machining 5-axis machining refers to the capability of a CNC machine to move a cutting tool or workpiece along five different axes simultaneously. These axes include the traditional linear X, Y, and Z axes, complemented by two rotational axes, often referred to as the A and B axes. This expanded range of motion facilitates the efficient and precise machining of complex geometries that would be challenging or impossible with conventional 3-axis systems. Key Features of 5-Axis Machining Increased Precision: 5-axis machines maintain optimal tool-to-part orientation, resulting in tighter tolerances and improved geometric accuracy. Complex Geometry Creation: The ability to approach the workpiece from multiple angles enables the production of intricate shapes and undercuts. Reduced Setup Times: Multiple faces of a part can often be machined in a single setup, minimizing non-cutting time and potential alignment errors. Improved Surface Quality: Continuous 5-axis interpolation allows for smoother toolpaths and more consistent chip loads, leading to superior surface finishes. The Impact of 5-Axis Machining in the Automotive Industry The automotive industry relies heavily on precision machining to produce components that meet stringent quality standards. 5-axis machining is particularly beneficial in this sector due to its ability to manufacture complex parts efficiently and accurately. Applications in Automotive Manufacturing Engine Components: The intricate geometries inside a car engine, such as optimized combustion chambers and complex cooling channels, are made possible through 5-axis machining. This technology allows manufacturers to pack more power into smaller spaces, enhancing engine performance. Gearboxes: Gearboxes are crucial for transmitting power from the engine to the wheels. 5-axis machining ensures that gears and shafts are manufactured with precision, resulting in reliable and efficient power transmission. Suspension Parts: Components like control arms and knuckles benefit from the precision of 5-axis machining, which ensures high strength and lightweight design, contributing to improved vehicle performance. Headlights and Taillights: The ability to carve complex shapes out of a solid block without layer lines makes 5-axis machining ideal for producing intricate headlight and taillight designs, enhancing both aesthetics and functionality. Advantages for Automotive Manufacturers Enhanced Reliability: High precision manufacturing ensures that automotive components are more reliable and dependable, reducing the likelihood of failures. Time-Efficient Production: The ability to produce small parts in extreme detail and carry out repetitive manufacturing efficiently has made automobiles more readily available in the market. Cost-Effective: While the initial setup cost of 5-axis systems is higher, the long-term benefits include shorter turnaround times and reduced labor intensity, leading to overall cost savings. Insights from Industry Leaders Leading manufacturers such as Hermle USA Inc. and FANUC have embraced 5-axis machining for its reliability and precision. Hermle’s automated machining centers, equipped with high-tech tool spindles and optimized chip management systems, cater to the automotive industry’s need for fast 3D work and complex machining tasks. FANUC’s 5-axis CNC systems offer advanced controls and error correction features, enhancing efficiency and accuracy in automotive component production. SEO Best Practices for 5-Axis Machining When discussing 5-axis machining, it’s essential to follow SEO best practices to ensure the content reaches a wider audience. Here are some tips: Meta Descriptions: Craft concise and compelling meta descriptions that summarize the article’s content and include the target keyword. Alt Text for Images: Use descriptive alt text for images related to 5-axis machining and automotive components to improve accessibility and search engine indexing. Internal/External Links: Incorporate links to authoritative sources, such as industry reports or manufacturer websites, to enhance credibility and provide additional information. Conclusion 5-axis machining is a game-changer in the automotive industry, offering precision, efficiency, and versatility in manufacturing complex components. As automotive manufacturers strive to meet evolving demands and enhance vehicle performance, the adoption of 5-axis machining continues to grow. By leveraging this technology, manufacturers can produce high-quality parts that contribute to safer, more reliable, and innovative vehicles. For those interested in exploring the capabilities of 5-axis machining or seeking expert services, consider reaching out to industry leaders-Prototek for tailored solutions. If you have any needs, contact us via email at Lynnyao@prototekparts.com or phone: +86-0792-86372550 Tags: Social Network: Recently Posts Online Help! +(86) 0791-86372550 WANT TO DISCUSS IN DETAIL Contact Our Consultant Now CONTACT NOW
Understanding Pump Covers in the Automotive Industry
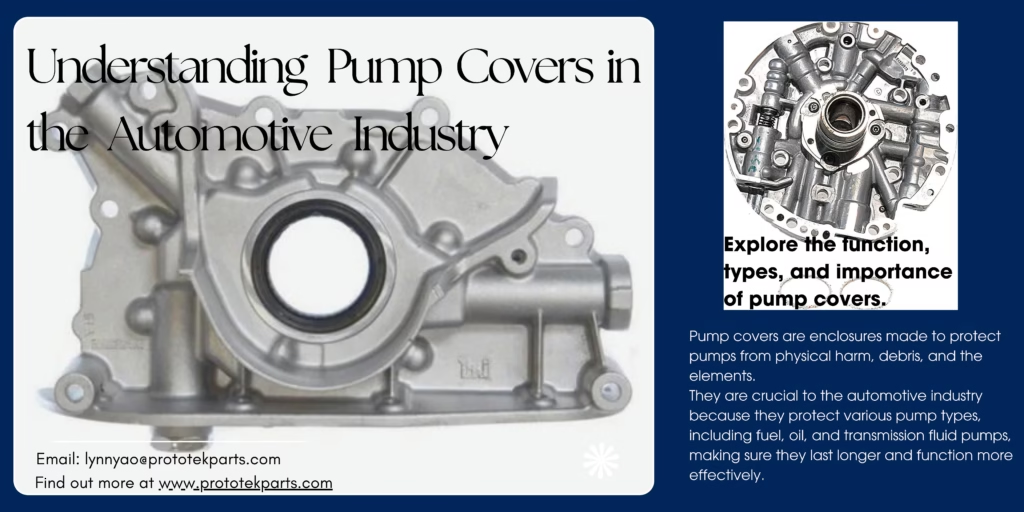
Understanding Pump Covers in the Automotive Industry Every part is essential to maintaining the longevity and optimum performance of cars in the dynamic automotive industry. Although they may not always be the most visible of these parts, pump covers are essential for safeguarding the many kinds of pumps found in automobiles. This article explores the types, advantages, and uses of pump covers in the automotive industry. Pump Covers: What Are They?Pump covers are enclosures made to protect pumps from physical harm, debris, and the elements. They are crucial to the automotive industry because they protect various pump types, including fuel, oil, and transmission fluid pumps, making sure they last longer and function more effectively. Pump Cover Types in the Automobile Sector 1. Oil Pump Covers Oil pump covers are essential to preserving the integrity of a car’s oil pump system. They keep impurities out of the oil system, which can cause wear and tear on engines. According to 4 Wheel Parts, oil pump covers are made to fit particular car models directly and are available for a variety of engines, including AMC V8 engines. 2. Covers for Transmission Fluid Pumps The purpose of transmission fluid pump covers is to safeguard the pumps that circulate transmission fluid within the transmission system. According to JEGS High Performance, these covers are crucial for stopping fluid leaks and preserving the transmission system’s effectiveness. They are available in a variety of designs, some of which have integrated coolers for improved efficiency. 3. Covers for fuel pumps Fuel pump covers are essential for protecting fuel pumps from harm and guaranteeing proper operation. Although they aren’t talked about as much as oil or transmission fluid pump covers, they are just as crucial to preserving the integrity of the car’s fuel system. Benefits of Using Pump Covers Protection from Environmental Elements Pump covers are primarily designed to protect pumps from harsh environmental conditions such as rain, snow, and dust. This protection is crucial for pumps installed outside the vehicle or in exposed areas. Grainger Industrial Supply emphasizes that pump covers allow pumps to operate outside without ambient debris or weather causing damage. Enhanced Pump Longevity By shielding pumps from contaminants and physical damage, pump covers extend the life of the pumps. This longevity translates into cost savings for vehicle owners, as it reduces the frequency of pump replacements and repairs. Improved Vehicle Performance Well-protected pumps operate more efficiently, contributing to the overall performance of the vehicle. For instance, a well-maintained oil pump ensures proper lubrication of engine components, leading to smoother engine operation. Cost-Effectiveness Investing in high-quality pump covers can lead to significant cost savings in the long run. By preventing damage and reducing maintenance needs, pump covers help vehicle owners avoid expensive repairs and replacements. Applications of Pump Covers in the Automotive Industry Commercial Vehicles In commercial vehicles, such as trucks and buses, pump covers are essential for protecting the larger and more exposed pumps. These vehicles often operate in harsh conditions, making pump covers a necessary component for maintaining reliability and performance. Off-Road Vehicles Off-road vehicles, including Jeeps and ATVs, are frequently exposed to extreme conditions, such as mud, water, and debris. Pump covers are crucial in these vehicles to ensure that pumps remain functional and protected during off-road excursions. Performance and Racing Vehicles In performance and racing vehicles, every component must operate at peak efficiency. Pump covers help maintain the optimal performance of pumps, contributing to the overall speed and reliability of these high-performance vehicles. Choosing the Right Pump Cover When selecting a pump cover, it is essential to consider factors such as material, fit, and compatibility with the specific pump type. High-quality materials, such as those used in ALCO Covers, provide excellent resistance to environmental elements, ensuring long-lasting protection. Material Considerations Durability: Choose materials that can withstand harsh conditions, such as industrial-strength vinyl or polyethylene. Weather Resistance: Opt for covers that offer protection against UV rays, rain, and extreme temperatures. Fit and Compatibility: Ensure the cover is designed to fit the specific pump model and application. In conclusion In the automotive industry, pump covers are a crucial but frequently disregarded component. They are essential to the upkeep and effectiveness of automobiles because they offer protection, increase longevity, and boost performance. Purchasing high-quality pump covers is a smart move that can result in significant cost savings and increased vehicle reliability, whether for performance, off-road, or commercial vehicles. Examining your options for pump covers is a good first step if you’re thinking about improving the longevity and performance of your car. Consider contacting industry suppliers or automotive specialists for additional information on choosing the best pump cover for your requirements. They can help you make an informed choice. In summary, pump covers are not merely add-ons; rather, they are vital parts that greatly enhance the general functionality and health of automotive systems. For smooth operation and a longer service life, make sure your car has the best pump covers installed. If you have any needs, contact us via email at Lynnyao@prototekparts.com or phone: +86-0792-86372550 Tags: Social Network: Recently Posts Online Help! +(86) 0791-86372550 WANT TO DISCUSS IN DETAIL Contact Our Consultant Now CONTACT NOW
A Comprehensive Guide to Control Arms
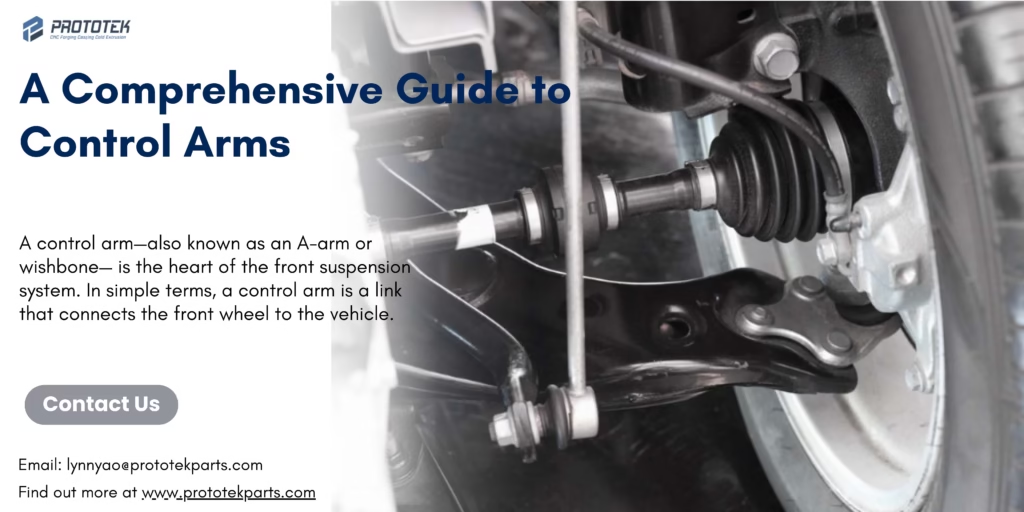
A Comprehensive Guide to Control Arms What is a control arm? A control arm—also known as an A-arm or wishbone— is the heart of the front suspension system. In simple terms, a control arm is a link that connects the front wheel to the vehicle. It is connected to the wheel assembly on one end and to the vehicle’s frame on the other. The upper control arm is connected to the top of the front wheel, the lower control arm is connected to the bottom of the front wheel, and both control arms are connected to the frame. If you have an independent rear suspension, the design is similar. Control arms help absorb shocks from the road and play a major role in vehicle steering, handling, and comfort. What are the types of control arm suspensions? The most common types of control arm suspensions are: Control arm suspension Strut suspension Control arm suspension is divided into the upper control arm & lower control arm Vehicles may have one or both, depending on the suspension type. Most front suspensions use lower control arms; double-wishbone systems use both. Also divided into OEM vs Aftermarket OEM (Original Equipment Manufacturer) arms offer perfect fitment and durability. Aftermarket control arms provide more flexible pricing and customization options. Strut designs have a lower control arm but no upper control arm. In a strut design, the strut acts as the upper control arm and is sometimes connected directly to the spindle or lower control arm. How do control arms work? Each control arm is connected to the vehicle frame via two control arm bushings. These bushings allow the control arm to move up and down. The other end of the control arm is connected to a steel spindle. The front wheel is bolted to the spindle. On vehicles not equipped with shock absorbers, the spindle is connected to the upper and lower control arms via ball joints. The ball joint is a steel ball enclosed in a steel sleeve that allows the spindle and front wheel to rotate from side to side and allows the wheel to move up and down along the road surface. Sandwiched between the control arm and the frame is a heavy steel coil spring that sits in a spring seat to support the weight of the vehicle and provide cushioning. To ensure perfect alignment of the control arms, bushings, and ball joints, some control arms have adjustable attachment points on the frame. If necessary, a technician can adjust the front end to ensure your vehicle stays straight on the road. Symptoms of a Bad Control Arm Timely replacement is crucial. Here are common signs of control arm failure: Clunking or knocking noises while driving over bumps Steering wheel vibration at higher speeds The vehicle is pulling to one side Uneven tire wear Loose or wandering steering If these symptoms appear, the control arm or its bushings/ball joints may be worn or damaged. When and Why to Replace Control Arms Control arms generally last 80,000–150,000 kilometers, depending on driving conditions and load. It’s important to: Replace them if any signs of cracking, bending, or bushing failure appear Check them during major suspension overhauls Use high-quality replacements that meet or exceed OEM specs to ensure safety How to diagnose control arm problems? A technician may diagnose control arm wear during a test drive of the vehicle. It may also be seen during a hop test, where the technician pushes hard on one corner of the vehicle to bounce the suspension and rotate the control arm. Because control arms determine the position and angle of the wheels, a damaged or bent control arm may be discovered during a four-wheel suspension alignment. How is a control arm replaced? The technician will first lift the vehicle in the air and then remove the wheel and tire before replacing the lower control arm. The lower control arm must be disconnected from the lower ball joint (which is attached to the steering knuckle) and the frame or body of the vehicle. The upper control arm is disconnected from the strut tower on the body and the top of the steering knuckle. Some control arms have built-in bushings, while others require bushings to be installed. When replacing a control arm, related parts such as the ball joint, tie rod joint, and stabilizer link are usually replaced at the same time. Control Arm Manufacturing: What Makes a Good Product? When sourcing control arms for export or wholesale, look for: Material Quality Forged aluminum or high-grade steel with proper corrosion resistance (e.g., electrophoretic coating) Precision Manufacturing CNC machining for exact tolerances Robotic welding or casting for consistent quality Ball Joint and Bushing Integration Durable rubber or polyurethane bushings Pre-assembled joints for easy installation Certifications & Testing ISO/TS 16949 certified production Fatigue and impact testing Salt spray resistance test (≥ 500 hours) How to Choose the Right Control Arm Supplier? Whether you’re importing for your retail brand or supplying to repair networks, consider: Product Range Does the supplier cover all major car models (e.g., Ford, Toyota, BMW, Hyundai)? MOQ & Lead Time Flexible minimum order quantity and fast delivery cycles Customization & Packaging Private label, barcode stickers, brand packaging Warranty & After-Sales Support At least a 12-month warranty is preferred for international buyers Factory Capabilities Visit or audit the factory to assess QC, automation level, and output capacity If you have any needs, contact us via email at Lynnyao@prototekparts.com or phone: +86-0792-86372550 Tags: Social Network: Recently Posts Online Help! +(86) 0791-86372550 WANT TO DISCUSS IN DETAIL Contact Our Consultant Now CONTACT NOW
Key Components in The Automotive Industry-Gas Springs
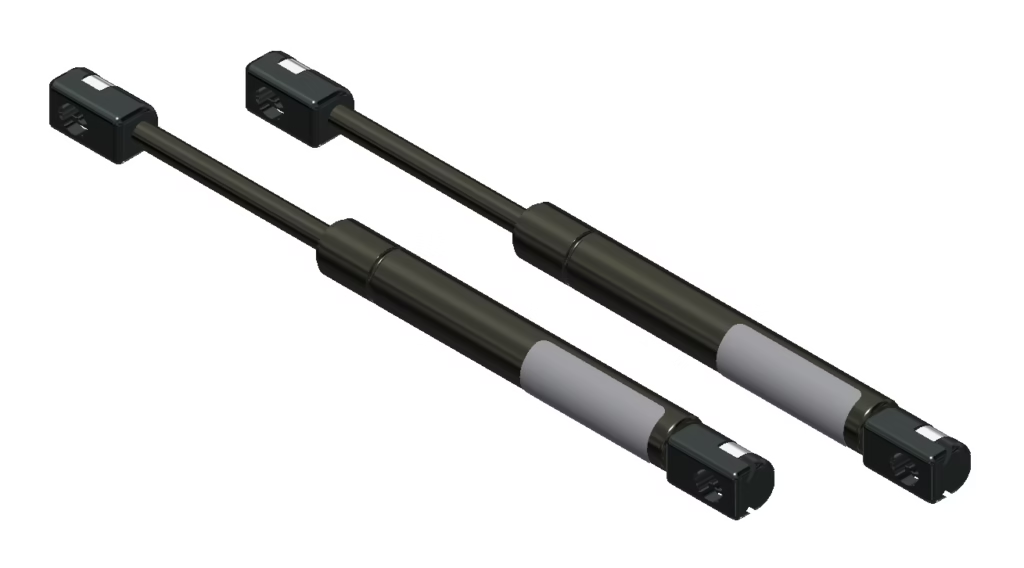
Key Components in The Automotive Industry-Gas Springs With the rapid development of the automotive industry, the demands for safety, comfort and intelligence have driven the innovation of many key components. Among them, gas springs, as a highly efficient energy storage and release device, play an increasingly important role in automotive design due to their unique performance advantages. From improving user experience to optimizing mechanical structure, gas springs are becoming an indispensable component in modern automotive engineering. Working principle and technical characteristics Gas springs are a mechanical device that stores energy by compressing inert gas (such as nitrogen). Its core consists of a sealed cylinder, a piston rod, and high-pressure gas. When an external force is applied, the piston rod compresses the gas to store energy; when the external force is released, the gas expands and pushes the piston rod to reset, achieving smooth motion control. Compared with traditional mechanical springs, gas springs have the following advantages: Controllable damping characteristics: By adjusting the gas pressure and valve design, the extension and retraction speed and force can be accurately controlled. Maintenance-free and long life: The sealed structure reduces wear and tear, and the service life can reach hundreds of thousands of cycles. Lightweight and compact: Under the same load, the gas spring is smaller in size, which helps to reduce the weight of the vehicle body. Silence and smoothness: There is no metal friction noise, and the movement process is smoother. Application scenarios In automobile design, the application of gas springs covers multiple dimensions from functionality to comfort: Improve convenience: intelligent control of doors, tailgates, and hoods Electric tailgate system: High-end SUVs and new energy vehicles widely use electric tailgates driven by gas springs, which can be opened and closed automatically by users through buttons, foot sensors or mobile phone apps. For example, the tailgate system of Tesla Model Y integrates intelligent gas springs, which supports custom opening angles and anti-pinch functions. Hood support: Gas springs replace traditional mechanical brackets, making the hood opening easier and reducing space. Enhance comfort: Optimization of seats and suspension systems Seat adjustment: The lumbar support, height adjustment, and folding functions of high-end car seats rely on gas springs to achieve stepless adjustment, providing personalized support for drivers. Active suspension system: Some models introduce gas springs in the suspension, which adjust the air pressure in real time to adapt to different road conditions and improve driving stability (such as Audi’s adaptive air suspension). Safety and functional expansion Collision energy absorption protection: In key parts such as the battery compartment cover of new energy vehicles, gas springs can be designed to quickly release energy during collisions to reduce the risk of structural damage. Roof and sunroof system: The folding roof of convertibles and the opening and closing mechanisms of panoramic sunroofs all require gas springs to provide stable power. Technology Trends: Intelligent and Sustainable Development As the automotive industry transforms towards electrification and intelligence, the technological iteration of gas springs is also accelerating: Electronic Gas Spring (E-Gas Spring) By integrating sensors and electronically controlled valves, gas springs can adjust the damping force in real time according to the vehicle status (such as vehicle speed and load). For example, in the scenario of autonomous driving, electronically controlled gas springs can cooperate with the automatic door opening and closing system to achieve more precise position control. Application of lightweight materials The use of materials such as aluminum alloy cylinders and carbon fiber piston rods further reduces weight. The lightweight tailgate system of Porsche Taycan achieves high energy consumption ratio through such technology. Environmental protection and recyclable design Regulations such as the EU ELV Directive promote the development of gas springs towards oil-free and detachable designs. Some manufacturers have launched recyclable seals and bio-based lubricants to reduce environmental pollution. Market prospects and challenges According to the report of Global Market Insights, the global automotive gas spring market size has exceeded US$1.5 billion in 2023 and is expected to grow at an average annual rate of 6.5% by 2030. However, the industry still needs to face the following challenges: Cost control: The R&D cost of high-end electronic control systems is high, and costs need to be reduced through large-scale production. Adaptability to extreme environments: High and low temperatures and dusty environments place higher demands on sealing performance. Standardization and compatibility: The installation interfaces and parameters of different models vary greatly, and it is necessary to promote the unification of industry standards. Although small, gas springs play a leveraging role in the “user experience revolution” of the automotive industry. From traditional mechanical components to parts of intelligent systems, their technological evolution not only reflects the refinement of engineering design, but also reflects the automotive industry’s unremitting pursuit of safety, comfort and sustainability. In the future, with breakthroughs in materials science and Internet of Things technology, gas springs may become an important element in defining the next generation of smart cars. 这是测试文本,单击 “编辑” 按钮更改此文本。 If you have any needs, contact us via email at Lynnyao@prototekparts.com or phone: +86-0792-86372550 Tags: Social Network: Recently Posts Online Help! +(86) 0791-86372550 WANT TO DISCUSS IN DETAIL Contact Our Consultant Now CONTACT NOW