What is Impact Extrusion
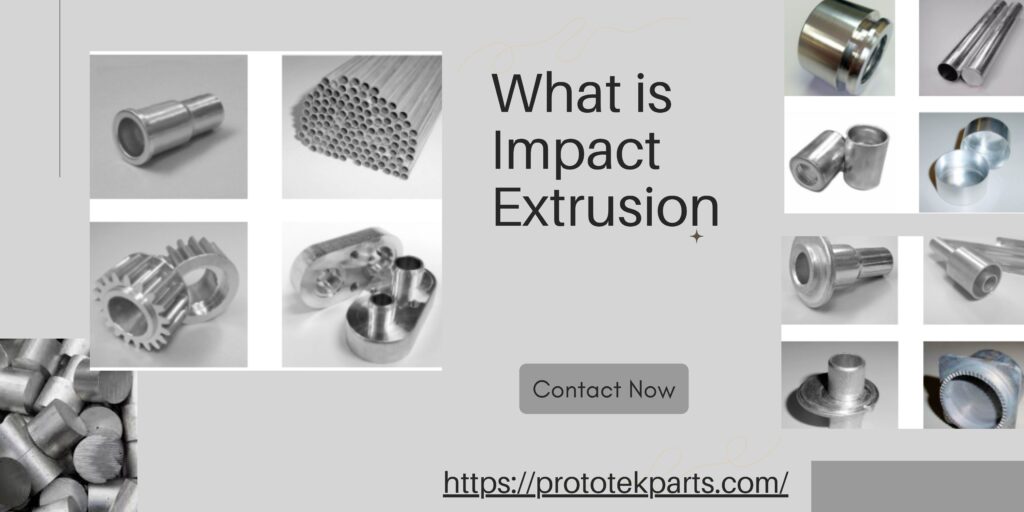
Blog Details Impact Extrusion is a metal processing technology that places a metal blank in a die. Under high-speed impact or pressure, the metal blank undergoes plastic deformation inside the die, ultimately forming the desired product shape. What is extrusion Extrusion is a metal-forming process typically used to create linear products with a fixed cross-sectional profile. It is accomplished by forcing metal (or other material) through a die with the desired solid or hollow cross-section. There are several benefits to extrusion. First, many shapes can be made through extrusion, often with very complex designs. It can also be used on otherwise brittle metals since the stresses involved are only compression and shear. The finished parts also tend to have an excellent surface finish. While impact extrusion is one method, there are a variety of extrusion options available to manufacturers. These include the standard hot and cold extrusion processes as well as friction extrusion. Another similar process is drawing, the only difference being that the metal is pulled through a die rather than pushed through it. What is the impact extrusion process? What distinguishes impact extrusion from other processes is that the workpiece is forced through an aperture at a much higher speed than other methods. The first step in the impact extrusion process is to take a block of metal and place it into a press. The press may be mechanical or hydraulic and can cycle up to 60 times per minute. The punch then pushes the block into the die at great speed, forcing the block’s metal to reshape around the punch while conforming to the outside of the die. This action controls the final shape of the workpiece, both inside and outside. Lubricants can be added as needed to facilitate this process. One strike is enough to form the block into its new shape, and then a reverse strike is used to eject the metal from the die. There are three types of impact extrusion operations. A forward strike is where the block is pushed into the die. A reverse strike forces the block around the punch. A combined strike is where the block is pushed into the die and around the punch simultaneously. It is important to note that the thickness of the workpiece is directly related to the gap between the punch and the die. This means that the thinner the workpiece, the tighter the tolerances. Also, as mentioned earlier, the surface finish of the finished piece will be better than the starting piece. The aluminum’s grain structure is refined during this work-hardening process, strengthening the metal in its new form. Metal extrusion can be subdivided into the following categories based on the direction of the extrusion flow and the medium through which the force is applied. Metal extrusion types Direct extrusion Indirect extrusion Hydrostatic extrusion Transverse or longitudinal extrusion Impact extrusion They can also be classified based on the operating temperature Hot extrusion Cold extrusion What are the advantages of impact extrusion? Impact extrusion is becoming increasingly popular, especially when processing aluminum alloys. As already noted, since it is a cold working technique, there is no need to preheat the workpiece, saving time and money while gaining the benefits of cold working. It is also faster because only one impact is required. The power of the punch mechanism is only required for one impact. Tooling costs tend to be lower, while the tooling itself lasts longer than other techniques. In terms of efficiency, the impact extrusion process has a high yield with minimal wasted material. Impact extrusion offers other advantages over other methods, including increased corrosion resistance, uniform grain alignment, and a lighter finished product. Additionally, the finished product requires little to no machining after processing. Which aluminum alloys are associated with impact extrusion? All aluminum alloys are well-suited for impact extrusion. Some of the advantages of aluminum alloys that make impact extrusion an excellent choice include its much lower density compared to steel, copper, nickel, and other metals. Aluminum is also non-toxic when it comes to food storage, and extrusion is widely used to produce food containers, such as beverage and food cans. Most of the aluminum alloys used in this process are in the 1000 series and 6000 series. What are some common impact extrusion applications? It is important to know that impact extrusions have certain limitations in terms of geometry. The finished product size needs to have a symmetrical cross-section, whether round, square, or oval; the base of the part must also be thicker than the sidewalls due to the nature of material flow in the process. Impact extrusions have a variety of uses. Common uses include pump components, pressure vessels, drive components, air conditioning components, fuel tank connectors, food and beverage cans, various cartridges, and tubes. If you have any needs, contact us via email at Lynnyao@prototekparts.com or phone: +86-0792-86372550 Tags: Social Network: Recently Posts Online Help! +(86) 0791-86372550 WANT TO DISCUSS IN DETAIL Contact Our Consultant Now CONTACT NOW
Everything You Need to Know About CNC Milling
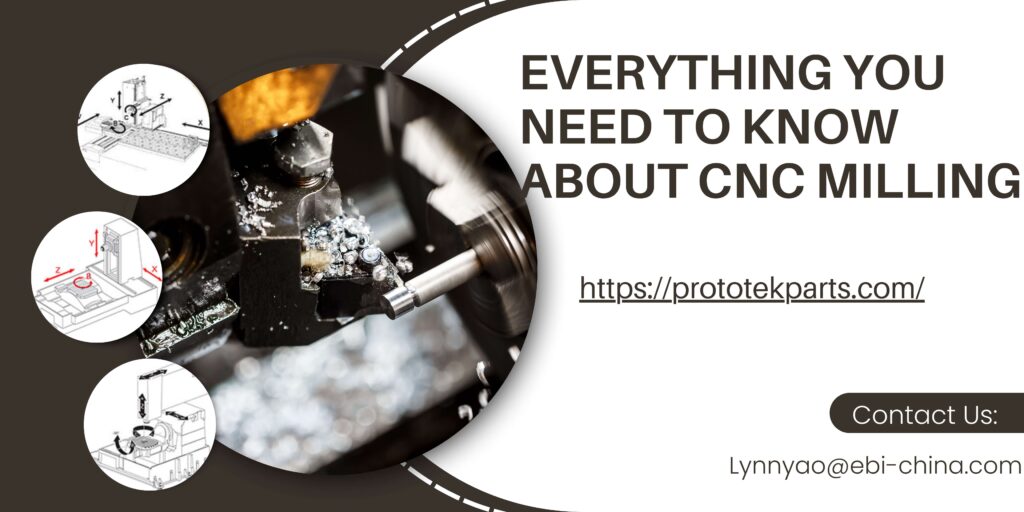
Blog Details CNC machining is Computer Numerical Control machining, the manufacturing process that uses computerized controls to control machines. CNC machining allows accurate and precise turning shaping and drilling of a variety of metals. In the following article, you will be focusing on the processes of CNC milling, and the advantages, as well as disadvantages of CNC milling. By understanding CNC milling, it will aid you in finding the best supplier. What is CNC Milling? CNC milling is the exact milling machining procedure that we have come to know and love but with the added benefit of computer-controlled. Milling is the cutting process of materials like plastic, metal, or wood with a tool that rotates. Every aspect, such as the cut toolpath, feed speed, and tool sequencing, is determined by the controls. Many different kinds of CNC milling machines permit various levels of complexity when it comes to cutting. The simplest models typically comprise three axes, however, more advanced models may contain up to five. They are utilized to create more intricate components. A variety of tools are available to help support different variations of the milling process. These range from traditional end mills and drill bits to specialty face milling and profile milling machines. With the many types of CNC cutters and mills virtually any design or shape can be created with the CNC milling equipment. The first commercially-available CNC milling device was designed during 1952 by Richard Kegg, in collaboration with J.F. Reintjes as well as his group from the Massachusetts Institute of Technology. Since then, a variety of advances regarding CNC milling technology have been made and include more advanced CNC milling machines, as well as automated. It makes use of a set of cutting tools that turn to make parts from blocks of material within one cycle. The material is taken off of the workpiece as the blade of the cutter comes into contact with the workpiece while it is rotating. When a milling cut is made, the workpiece is securely held in place while the cutter begins to remove the material. The exact paths of the cutter cutting depths, cut lengths, the XYZ and axis movement as well as spindle RPM all have previously been added to the control software. The process is utilized in a variety of industries, including automotive aerospace and construction, agriculture electronic, consumer goods. To create a variety of components, including components for engines and landing gears, and tanks for fuel in airplanes and surgical devices like surgical scalpels as well as implants. What is the CNC milling machine’s function? Step 1: Design a CAD model Machinists utilize design software to design a CAD-based model of the specific part. Step 2: convert your CAD model to a CNC machine Then, you can transfer the CAD model to The CAM CNC system, which is a computer-aided production. If you do it correctly you’ll have an array of digital instructions that instruct what the machine it should do, also known as G-code. G-code commands allow machines to determine which direction to travel and how quickly to complete it to produce a specific component. Step 3: Configure the CNC milling machine Attach the block of material or workpiece to the machine’s bed. Using metrology instruments and touch probes, ensure that it’s aligned properly. Install the machine’s spindle and follow the steps to set up your milling equipment. Step 4 Start the milling process Launch the program to begin to begin the CNC cutting process. The special cutting tools spin at a high speed or at a set number of revolutions per minute to remove materials from the workpiece until it is able to accurately reproduce the part you’re looking for. What are the 3-, 4– and 5-axis milling machines? 3-axis milling machines permit the cutting tool to move and subtract components through the Z, X, and Y Axes. This type of machining is most well-known due to its low initial cost. It is also employed to make basic parts that have a simple geometrical design. A 4-axis milling machine comes with all the advantages of a three-axis milling machine plus another axis. Additionally, it permits the workpiece to rotate to cut on the A-axis. This is especially helpful for parts that need to be cut in a circle, or the edge of an object. The 5-axis milling machines allow for the movement of three linear axes, as well as the turning of the machine’s head as well as the tool head. These are five axes in one. It produces products with complicated geometries like aerospace products including titanium parts, medical devices, and gas machine components. Since it can perform multidimensional rotation that eliminates the necessity of multiple setups and permits one-step machining that is quicker and more efficient. CNC Milling Methods Many different milling tools can be used to create components. First was plain milling, a technique that cuts flat surfaces that are parallel to the rotation of the tool. Since its initial appearance on the scene of manufacturing, CNC machining has led to various other types of milling being created. This includes: Face milling: It cuts materials to form a surface that is perpendicular to the rotation axis of the cutting tool. Angulous Milling: removes material from the plane surface by making an angle. Form Milling: It also cuts material however this time, to create an irregular surface (i.e. curvatures, etc.). Gang Milling The method used is capable of increasing the speed of production since it employs two cutters, and sometimes more than one, to cut the material. Surface Milling: Used to remove material from the surface of a workpiece to flatten or form a specific shape. Mainly used for machining planes or contour edges, usually using a flat milling cutter or an end mill. Suitable for machining simpler workpiece surfaces such as planes, grooves, holes, etc. Profile Milling: Used to cut on the surface of a workpiece according to a specific contour or curve shape. Can be used to machine complex contours or curve shapes, usually requiring a specific tool to be designed according to the contour of the workpiece. Commonly used for machining
What is Cold Forging and How Does It Work?
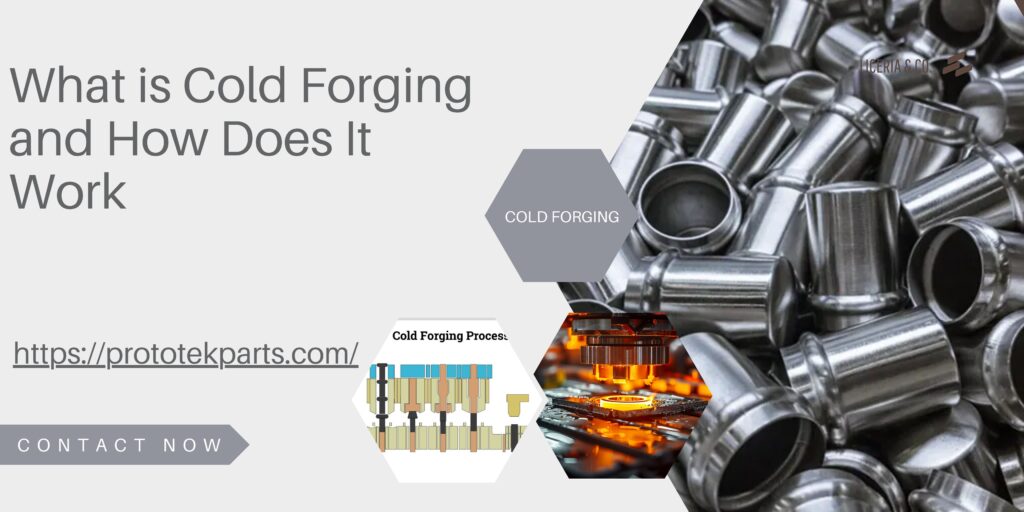
Blog Details Cold forging is a metal shaping technique where metal bar stock is placed into a die and pressed into another closed die at ambient temperature or lower than the metal’s austenite temperature. This process is highly efficient and cost-effective for producing large quantities of components. Forging methods can be categorized into cold, warm, and hot forging, all forgings involve tools such as hammers, dies, or presses to shape and deform the metal. It should be distinguished from machining and casting, as it results in a product that is stronger and of superior quality. Unlike warm or hot forging, cold forging manipulates the metal at room temperature, using localized compressive forces to achieve the desired shape. Depending on the part’s design, the metal may be subjected to multiple dies or repeated strikes to reach the final form. How Does It Work? Cold forging offers significant cost savings due to reduced labor and the elimination of additional processing. Parts can be manufactured at high speeds – up to 1000 units an hour! – which further lowers per-piece costs. This process entails feeding metal coils into machines that perform the work quickly and efficiently. The Cold Forging Process Step 1: Lubricant Before the forging process begins, the workpiece is often coated with a lubricant. This helps to prevent adhesion to the die and maintains a lower temperature during forming, as deformation can generate heat ranging from 250°C to 450°C. The application of lubricants varies by manufacturer and their specific methods, and they are not universally employed in all processes. Step 2: Feeding the Metal Coil The metal coil is introduced into the forging machine, positioned above a die that is shaped to form the final part. This die typically consists of two segments: one attached to the hammer, and the other positioned beneath the workpiece. The hammer, which is the upper part of the mechanism, delivers the force necessary to shape and deform the metal. Step 3: The Stroke Three primary mechanisms can be used to strike the workpiece: hydraulic, pneumatic, or mechanical. Each method employs a shaft with an attached hammer that descends with significant force onto the workpiece to achieve the intended shape. This process occurs in milliseconds. Occasionally, multiple hammer strikes may be necessary in rapid succession to achieve the precise contour and dimensions required. Step 4: Part Removal The method of part removal varies with the specific process used. In contemporary manufacturing, automation often handles this task through conveyors or robotic arms. This approach reduces costs by eliminating the need for manual material handling. Step 5: After Shaping The approach to handling parts post-forging varies depending on their complexity. Single-die, single-stroke parts are typically trimmed and forwarded to shipping directly. Additional die processes are required to incorporate various features for more intricate components. What are the benefits of cold forging? 1. High Precision: Cold forging allows the production of parts with tight tolerances and complex geometries. The process requires precise control over die dimensions, punch forces, material flow and die alignment; ultimately producing components requiring minimal additional machining work – an attribute particularly valuable in industries where exact specifications are key, such as automotive and aerospace manufacturing. 2. Superior Surface Finish: Compared to hot forging or other metal forming methods, cold forging typically produces parts with excellent surface smoothness and finish. The absence of high temperatures reduces oxidation and scale formation on the metal surface, eliminating or minimizing the need for secondary finishing processes like grinding or polishing. 3. Material Savings: Cold forging has long been recognized for its efficient material utilization efficiency. This process involves shaping metal without significant material loss, making it an attractive solution when cost and waste reduction are top priorities. Manufacturers can maximize material savings by designing dies and processes that minimize scrap generation for enhanced cost-effectiveness and sustainability in production. 4. Improved Mechanical Properties: Cold forging allows for the production of parts with tight tolerances and complex geometries, using precise control over die dimensions, punch forces, material flow, and material removal – often with minimal additional machining required post-production. 5. Design Flexibility: Cold forging allows for the production of complex shapes and configurations that may be difficult or costly to achieve through other manufacturing methods. The versatility of cold forging dies enables the creation of various part geometries, including asymmetrical and multi-dimensional forms, thereby expanding design possibilities for engineers and product designers. 6. Cost-Effectiveness: Despite requiring specialized equipment and tooling, cold forging offers long-term cost benefits due to reduced material waste, lower energy consumption (compared to hot forging), and minimal need for secondary operations. 7. Environmental Benefits: Cold forging aligns with sustainable manufacturing practices by minimizing material waste, reducing energy consumption, and lowering carbon emissions associated with heat-intensive processes. Disadvantages of Cold Forging Cold forging is not without its drawbacks. As with any production method, it has its limitations and constraints that should be considered before selecting it for your next project. 1. Only simple shapes and designs in high volumes can be produced. Unique, unusual, or intricate patterns cannot be cold-forged. Deformation levels and shaping grades are limited, and cold-forged metals have less ductility. 2. The alteration of the grain structure of a metal gives it extra strength but may produce residual stress. 3. Certain methods of cold forging require heat treatments to eliminate possible cracks or creep hardening. 4. There is a limitation to the types of metals that can be cold forged. Ones with low ductility and sensitivity to strain hardening should not be chosen since they lose their ductility and will break under tensile stress. Only metals with a hardness of HRC 44 on the Rockwell scale can be used. 5. Production processes require a great deal of force to produce the necessary compressive force and pressure. Though the addition of hydraulics and pneumatics has decreased the size of the equipment, it has not eliminated the amount of power the machines consume. 6. Tools, dies, and molds have to be specially designed as well as sturdy and strong enough to be
Aluminum Precision CNC Machining Services
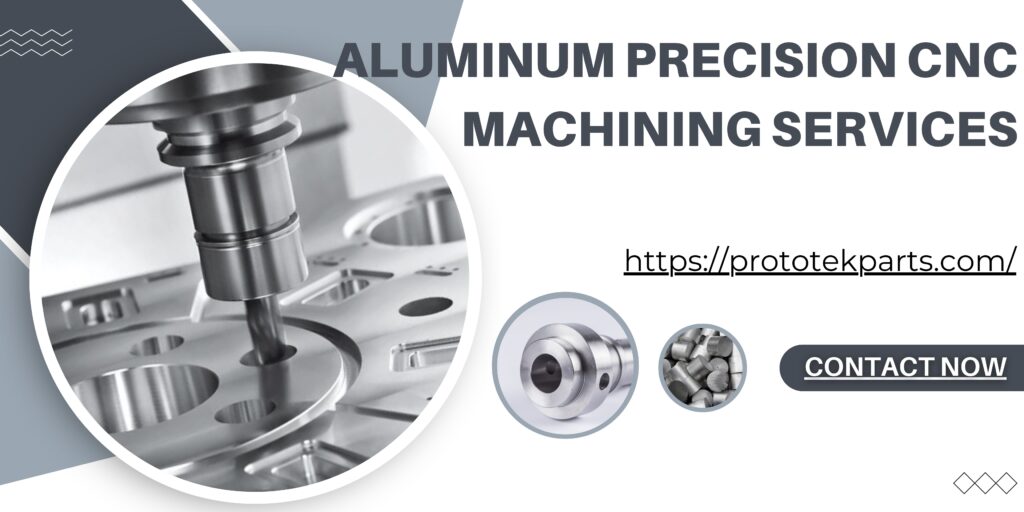
Blog Details Aluminum precision CNC machining is an essential service in the manufacturing industry, providing companies with the ability to create highly accurate and complex components from aluminum. This process leverages advanced technology to produce parts that meet exact specifications, making it a preferred choice for various applications across multiple industries. In this article, we will explore the benefits, processes, applications, and considerations related to aluminum precision CNC machining services. Understanding CNC Machining CNC machining is the manufacturing process of subtractive, where computers are controlled to carry out operations like milling, turning drill, and grinding to produce components using the raw materials. It is important to note that the word “CNC” stands for “Computer Numerical Control,” which means that the machines work according to pre-programmed instructions that ensure accuracy and reliability. Aluminum is one of the most commonly machined materials due to its lightweight, strength, and resistance to corrosion. Its excellent machinability makes it an ideal candidate for precision CNC machining, allowing manufacturers to produce intricate parts with tight tolerances. Advantages of Aluminum in CNC Machining Lightweight and Strong: Aluminum’s high strength-to-weight ratio makes it an ideal choice for applications where weight reduction is crucial, such as in the aerospace and automotive industries. Corrosion Resistance: Aluminum naturally forms a protective oxide layer, providing excellent resistance to corrosion, and making it suitable for outdoor and harsh environments. Excellent Thermal and Electrical Conductivity: These properties make aluminum a popular choice for components in electronics and heat exchange systems. Versatile Applications: Aluminum can be easily alloyed with other elements, enhancing its properties and broadening its range of applications. Cost-Effectiveness: While aluminum can be more expensive than some other materials, its durability and low maintenance costs often lead to lower overall production costs. The CNC Machining Process for Aluminum The aluminum CNC machining process involves several key steps: Design and CAD Modeling: The first step is to create a detailed design of the component using Computer-Aided Design (CAD) software. This design serves as the blueprint for the CNC machining process. Programming the CNC Machine: Once the design is finalized, it is converted into a format that the CNC machine can understand, usually G-code. This code includes all the instructions necessary for the machine to create the part. Material Selection: Selecting the appropriate aluminum alloy is crucial. Different alloys possess different mechanical properties, so choosing one that aligns with the project’s requirements is essential. Machining Operations: The CNC machine executes the programmed instructions, performing various operations such as milling, turning, drilling, and tapping. Advanced CNC machines can handle multiple operations in a single setup, enhancing efficiency. Quality Control: Throughout the machining process, quality control measures are implemented to ensure that the components meet specified tolerances and surface finish requirements. Techniques like Coordinate Measuring Machines (CMM) may be used for precise measurements. Finishing: After machining, components may undergo finishing processes, such as anodizing, polishing, or coating, to enhance their appearance and properties. Applications of Aluminum Precision CNC Machining Aluminum precision CNC machining is utilized across a wide range of industries. Some notable applications include: Aerospace: Aluminum parts are widely used in aircraft due to their lightweight nature and strength. Components such as brackets, housings, and structural elements are often produced using CNC machining. Automotive: The automotive industry relies on aluminum for engine parts, transmission housings, and chassis components, contributing to overall vehicle weight reduction and fuel efficiency. Electronics: Aluminum is used in the manufacturing of enclosures, heat sinks, and other electronic components due to its excellent thermal conductivity and aesthetic appeal. Medical Devices: In the medical field, precision-engineered aluminum parts are critical for instruments, implants, and devices that require high reliability and biocompatibility. Consumer Products: Many consumer products, such as appliances and sporting goods, benefit from aluminum components that combine functionality with an appealing finish. Factors to Consider When Choosing a CNC Machining Service When selecting a CNC machining service for aluminum parts, several factors should be taken into account: Experience and Expertise: Choose a supplier with extensive experience in aluminum machining. Their knowledge of materials, tooling, and machining techniques is crucial for achieving high-quality results. Capabilities and Equipment: Ensure that the machining service has state-of-the-art CNC machines capable of handling the complexity and volume of your projects. Quality Assurance: Inquire about the supplier’s quality control processes. A reliable service should have stringent quality checks in place to guarantee precision and consistency. Lead Times: Discuss lead times upfront to ensure that the supplier can meet your project deadlines. Timely delivery is essential in maintaining production schedules. Customer Support: Good communication is vital throughout the project. Choose a supplier that offers responsive customer support to address any concerns or questions. Cost-Effectiveness: While price should not be the only consideration, it is important to evaluate the overall value offered by the supplier, including quality, service, and delivery times. The Future of Aluminum Precision CNC Machining The future of aluminum precision CNC machining appears optimistic, driven by technological innovations and increased demand across various sectors. CNC technologies like automation and artificial intelligence are improving machining efficiency and precision while an increased focus on lightweight materials in industries like aerospace and automotive is likely to further bolster demand for aluminum machining services. Sustainability is becoming an increasingly important consideration in manufacturing. Aluminum can be recycled, while using advanced CNC machining techniques can minimize waste and energy usage – aligning perfectly with environmentally friendly practices. Aluminum precision CNC machining services play an invaluable role in modern manufacturing, offering unparalleled precision and versatility. By understanding its benefits, processes, and applications businesses can make informed decisions about their manufacturing needs. As technology evolves aluminum machining will continue to expand in scope allowing greater opportunities for innovation and efficiency across various industries. Whether you are in aerospace, automotive, electronics, or any other field, partnering with a reliable CNC machining service can help you achieve your production goals while ensuring the highest quality standards. If you have any needs for the aluminum precision custom part, contact Prototek by Lynnyao@prototekparts.com or phone: +86-0792-86372550. You will feel deserved
Finding a Reliable Custom CNC Supplier
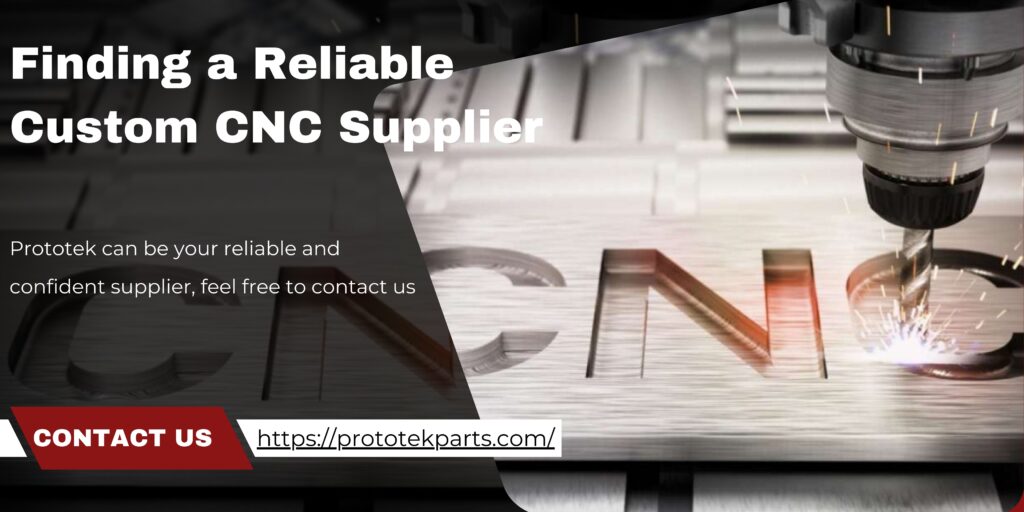
Blog Details The need for precision-engineered components is growing in the current fast-paced manufacturing industry. Nowadays, a custom CNC (Computer Numerical Control) is an essential process across a variety of industries including aerospace, electronics, automotive medical devices, and more. However, any success you can get from your project is contingent on locating an experienced custom CNC provider. In this article, you will through the essential steps to locate a trustworthy supplier that meets your needs. Understanding Your Requirements: Before you start your search for a custom CNC supplier, it’s crucial to have a clear understanding of your project requirements. Consider the following factors: Materials: Determine the materials you need for your components. CNC machining can work with various materials, including metals (aluminum, steel, titanium) and plastics (ABS, nylon, polycarbonate). Tolerances: Understand the precision required for your components. Different suppliers have varying capabilities in achieving tight tolerances. Volume: Consider whether you need a small batch of prototypes or large-scale production runs. Some suppliers specialize in one or the other. Lead Time: Assess your timeline. Ensure the supplier can meet your deadlines without compromising quality. Design Complexity: Evaluate the complexity of your designs. Some suppliers may excel in intricate parts while others may be better suited for simpler components. Researching Potential Suppliers Once you have a clear understanding of your requirements, the next step is to research potential suppliers. Here are some effective strategies: Online Directories and Marketplaces: Websites like ThomasNet, Maker’s Row, and Alibaba can help you find CNC suppliers. Look for those with positive reviews and a robust portfolio. Industry Associations: Many industries have associations that provide resources and directories for reliable suppliers. Check if your industry has such a network. Trade Shows and Exhibitions: Attending industry-specific trade shows is an excellent way to meet suppliers in person. You can see their products firsthand and discuss your needs directly. Networking: Reach out to peers in your industry for recommendations. Personal referrals can lead you to suppliers that others have had positive experiences with. Social Media and Forums: Platforms like LinkedIn and industry-specific forums can be valuable for gathering insights and recommendations about CNC suppliers. Evaluating Suppliers Once you have a shortlist of potential suppliers, it’s essential to evaluate them to ensure they meet your standards: Experience and Expertise: Look for suppliers with extensive experience in CNC machining. Check their history and the industries they have served. Certifications: Ensure the supplier holds relevant certifications, such as ISO 9001, which indicates a commitment to quality management systems. Capabilities: Inquire about their equipment and technology. Advanced machinery and software can improve precision and efficiency. Quality Control Processes: Ask about their quality assurance processes. A reliable supplier should have a robust system for inspecting and testing components before delivery. Case Studies and References: Request case studies or references from previous clients. This can give you insight into their work quality and customer service. Communicating Your Needs Effective communication is vital for a successful partnership with a CNC supplier. When discussing your project, be clear and concise about your requirements: Provide Detailed Specifications: Share detailed drawings and specifications, including dimensions, tolerances, and surface finishes. Set Expectations: Communicate your expectations regarding timelines, budgets, and quality. Discuss Problem-Solving: Gauge how the supplier approaches challenges. A proactive supplier will provide solutions and suggestions to enhance your project. Requesting Quotes and Prototypes Once you have narrowed down your list of potential suppliers, request quotes. Consider the following when evaluating quotes: Pricing: While cost is important, the cheapest option may not always be the best. Consider the overall value, including quality and service. Turnaround Times: Compare the lead times provided by each supplier. Ensure they align with your project timeline. Prototype Development: Ask if they offer prototype services. Testing a prototype before full-scale production can help identify issues early on. Building a Long-Term Relationship Once you have chosen a supplier, focus on building a long-term relationship. A reliable custom CNC supplier can be a valuable partner in your manufacturing process. Here are some tips for fostering a successful collaboration: Maintain Open Communication: Keep the lines of communication open. Regular updates and feedback can help resolve issues promptly. Evaluate Performance: Continuously assess the supplier’s performance against your expectations. Provide constructive feedback and recognize their efforts. Find new opportunities: If your project is growing think about discussing possibilities with the supplier. They might have insight or capabilities that can be beneficial to your future ventures. Locating a trustworthy custom CNC supplier takes careful research and analysis. By analyzing your requirements conducting thorough assessments and maintaining clear communications, you can create a partnership that will improve the efficiency of your manufacturing processes. Reliable partners can meet your immediate and long-term needs in an increasingly competitive market. By taking the appropriate steps, you will discover custom CNC machine machining and select services provided by companies that match up well with your vision and objectives. Prototek can be your reliable and confident supplier, feel free to contact us by Lynnyao@prototekparts.com or phone: +86-0792-86372550 Tags: Social Network: Recently Posts Online Help! +(86) 0791-86372550 WANT TO DISCUSS IN DETAIL Contact Our Consultant Now CONTACT NOW
Steel CNC Machining – A Comprehensive Guide
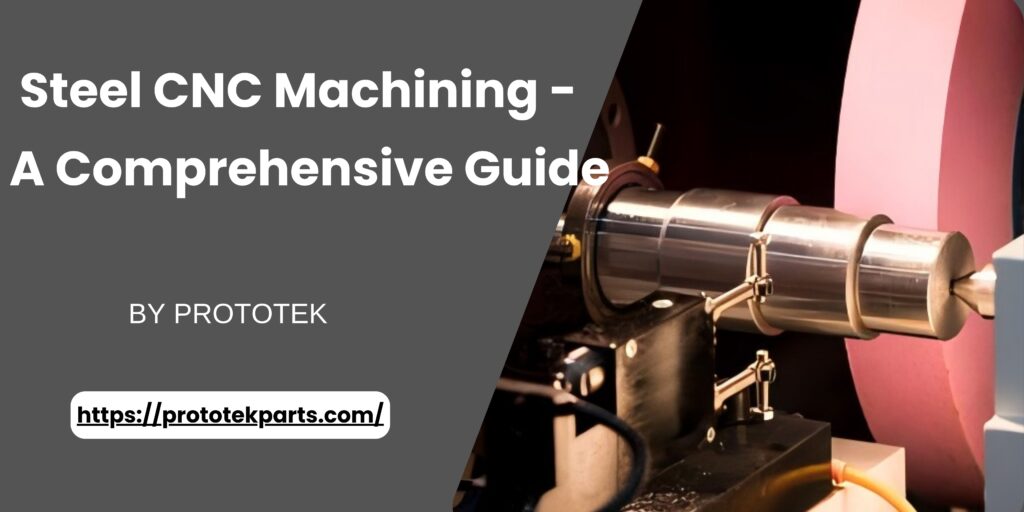
Blog Details In the modern manufacturing industry, precision machining has become crucial for enhancing production efficiency and product quality. Steel CNC (Computer Numerical Control) machining, a key technology in this domain, not only meets complex design requirements but also improves precision and consistency in production processes. Prototek stands out as a leading company in steel CNC machining, offering exceptional technology and services to help clients achieve their business goals. This article provides an in-depth look at how Prototek utilizes steel CNC machining to drive success. Prototek’s Core Competencies High Precision Machining At Prototek, we are committed to delivering world-class steel CNC machining services. We utilize state-of-the-art CNC equipment and technologies to ensure that each step of machining is completed with absolute precision. If you are dealing with intricate geometric forms or intricate details the equipment we use is specifically designed to operate with a high degree of precision. Prototek’s equipment is equipped with ultra-precision sensors and control systems to ensure that every part is in line with strict specifications. Efficient Production Capabilities We understand that time equates to productivity. Our CNC machines not only operate at high speeds but also feature automation capabilities, allowing for extended periods of continuous operation. This high-efficiency production capability helps us meet large-scale manufacturing needs and swiftly respond to urgent orders, ensuring timely delivery of services. Flexible Machining Capabilities Prototek offers highly flexible CNC machining services, capable of adjusting programming and machining settings according to varying customer needs. Whether it’s small-batch production or large-scale manufacturing, we can quickly adapt production plans to meet personalized requirements. We focus on providing customized solutions to ensure optimal results for every project. Advantages of Choosing Prototek 1. Extensive Experience With years of experience in steel CNC machining, Prototek’s team comprises skilled engineers and technicians. We are well-versed in the properties of various steel types and can offer professional advice and solutions based on different industry standards. Whether it’s the automotive industry, aerospace, or construction, our track record of successful projects supports our expertise. 2. Advanced Technology Prototek remains at the forefront of technology by continually investing in the latest CNC equipment and innovations. Our equipment includes high-speed and precision milling, turning, and grinding machines that can handle an array of complicated steel-related machining tasks. We are also investigating the latest technologies, like automated control and intelligent manufacturing to improve the efficiency and quality of our machines. 3. Rigorous Quality Control Quality control is a top priority at Prototek. Every machining process is subject to strict tests and inspections for ensuring that finished products are of the highest standards of quality. From selecting the material to the last inspection extensive quality management system guarantees that the needs of every customer are completely satisfied. 4. Exceptional Customer Service Prototek is dedicated to providing an outstanding customer service experience. Our customer service team tracks your project progress closely, communicates promptly, and addresses any issues. We believe that a close partnership with our clients allows us to comprehend their needs and provide specific services. From technical advice to support after sales, we’re committed to offering comprehensive support. Application Areas 1. Automotive Industry In automotive manufacturing, precision and durability are paramount. Prototek offers machining services for various steel components utilized for automotive manufacturing. These include components for the chassis, engine parts, and body structure. Prototek’s precision and efficiency manufacturing capabilities allow our clients to increase efficiency and lower costs. 2. Aerospace The aerospace industry demands stringent standards for materials and components. Prototek offers machining services for high-strength, high-temperature steel parts used in aerospace applications. Which ensures that each component meets rigorous aerospace standards, and contributes to the safety and performance of aircraft and spacecraft. 3. Construction In construction, the strength and stability of structural components are crucial. Prototek provides machining services for various construction steel components, including support beams, columns, and connectors. Our technology ensures the high safety and reliability of building structures. 4. Electronics Components in electronic devices require precise machining to ensure proper functioning. Prototek provides machining services for steel parts used in electronic devices, such as enclosures, brackets, and heat sinks. Our technology guarantees that each component meets high standards for performance and reliability. Future Outlook Prototek is committed to ongoing technological innovation and development. In the future, we will continue to invest in advanced machining technologies and equipment, exploring more efficient production methods to meet evolving market demands. We are also actively pursuing the application of intelligent manufacturing and automated control to further enhance machining efficiency and quality. Conclusion Prototek has established a strong reputation in the field of steel CNC machining through its exceptional technology and services. From high precision and efficient production to outstanding customer support, we are dedicated to providing the best solutions for our clients. Partnering with Prototek allows you to benefit from top-quality machining services, helping your business stand out in a competitive market. We look forward to collaborating with you and achieving greater success together! If you have any needs, contact us via email at Lynnyao@prototekparts.com or phone: +86-0792-86372550 Tags: Social Network: Recently Posts Online Help! +(86) 0791-86372550 WANT TO DISCUSS IN DETAIL Contact Our Consultant Now CONTACT NOW